Classical Grignard reaction | |||||||||||
---|---|---|---|---|---|---|---|---|---|---|---|
Named after | Victor Grignard | ||||||||||
Reaction type | Coupling reaction | ||||||||||
Reaction | |||||||||||
| |||||||||||
Identifiers | |||||||||||
Organic Chemistry Portal | grignard-reaction | ||||||||||
RSC ontology ID | RXNO:0000014 | ||||||||||
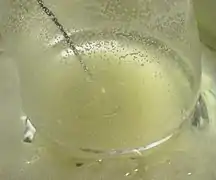
The Grignard reaction (French: [ɡʁiɲaʁ]) is an organometallic chemical reaction in which, according to the classical definition, carbon alkyl, allyl, vinyl, or aryl magnesium halides (Grignard reagent) are added to the carbonyl groups of either an aldehyde or ketone under anhydrous conditions.[1][2][3] This reaction is important for the formation of carbon–carbon bonds.[4][5]
(R2 or R3 could be a hydrogen)

Grignard reactions and reagents were discovered by and are named after the French chemist François Auguste Victor Grignard (University of Nancy, France), who published it in 1900[6] and was awarded the 1912 Nobel Prize in Chemistry for this work.[7] The reaction of an organic halide with magnesium is not a Grignard reaction, but provides a Grignard reagent.[8] Other variations of the Grignard reagent have been discovered to improve the chemoselectivity of the Grignard reaction which include but are not limited to: Turbo-Grignards, Organocerium reagents, and Organocuprate (Gilman) reagents.
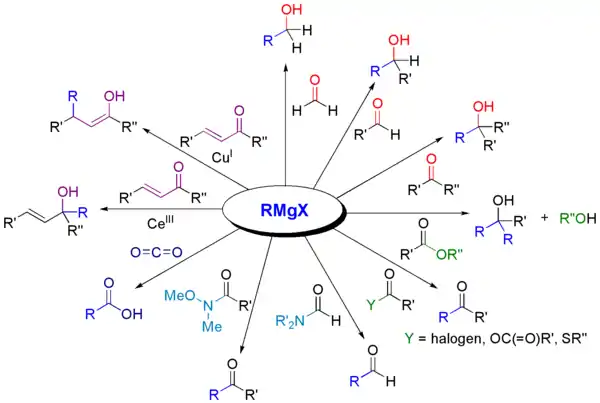
Reaction mechanism
Because carbon is more electronegative than magnesium, the carbon attached to magnesium acts as a nucleophile and attacks the electrophilic carbon atom in the polar bond of a carbonyl group. The addition of the Grignard reagent to the carbonyl group typically proceeds through a six-membered ring transition state, as shown below.[9]
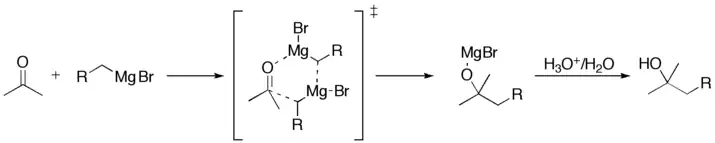
Based on detection of radical coupling side products, an alternative single electron transfer (SET) mechanism that involves the initial formation of a ketyl radical intermediate has also been proposed.[10] A recent computational study suggests that the operative mechanism (polar vs. radical) is substrate dependent, with the reduction potential of the carbonyl compound serving as a key parameter.[11]
Grignard Reaction Conditions
The Grignard reaction must be completed under anhydrous conditions.[3] Otherwise, the reaction will fail because the Grignard reagent will act as a base rather than a nucleophile and pick up a labile proton rather than attacking the electrophilic site. This will result in no formation of the desired product as the R-group of the Grignard reagent will become protonated while the MgX portion will stabilize the deprotonated species.
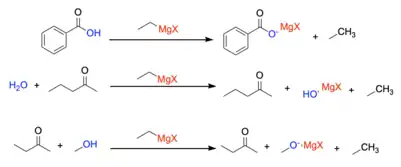
To prevent this, Grignard reactions are completed in an inert atmosphere to remove all water from the reaction flask and ensure that the desired product is formed.[12] Additionally, if there are acidic protons in the starting material as shown in the figure on the right, one can overcome this by protecting the acidic site of the reactant by turning it into an ether, or a silyl ether to eliminate the labile proton from solution prior to the Grignard reaction.
Grignard-like reactions using more chemoselective reagents
The classical Grignard reaction typically refers only to the reaction between a ketone or aldehyde group and a Grignard reagent to form a primary or tertiary alcohol.[6] However, some chemists understand the definition to mean all reactions of electrophilic molecules with Grignard reagents.[13]
Therefore, there is some dispute about the modern definition of the Grignard reaction.
March's Advanced Organic Chemistry, a reputable graduate level text, defines a Grignard reaction as "The addition of Grignard reagents to aldehydes and ketones...".[1] The IUPAC Goldbook, a similarly reputable text, does not address either side.[8] In the Merck Index, published online by the Royal Society of Chemistry, the classical definition is acknowledged, followed by "A more modern interpretation extends the scope of the reaction to include the addition of Grignard reagents to a wide variety of electrophilic substrates."[13] This variety of definitions illustrates that there is some dispute within the chemistry community about the definition of a Grignard reaction.
Shown below are some reactions involving Grignard reagents, but themselves are not classically understood as Grignard reactions.
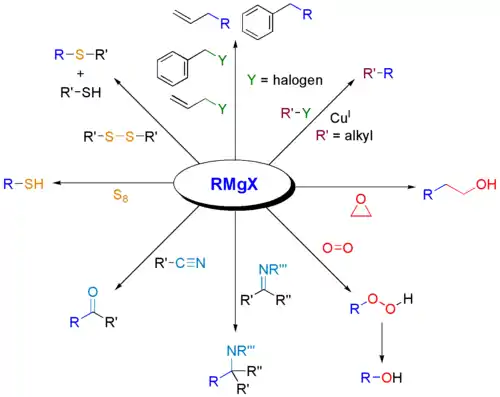
Turbo-Grignards
Turbo-Grignards can be used to form complex aryl and heteroaryl Grignard reagents.[14] The formation of Turbo-Grignards are chemoselective. Esters, amides, and nitriles do not react with the Turbo-Grignard reagent.[14]
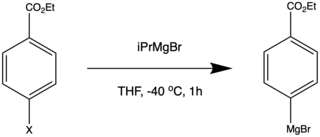
Organoceruim Reagents
Organocerium reagents are a variation of the classic Grignard reagent which selectively adds 1,2 to conjugated Ketones and Aldehydes.[15] Organocerium reagents favour direct addition to α,β-unsaturated carbonyl groups.[15] This preference for direct addition is due to the oxophilicity of the cerium reagent which activates the carbonyl for nucleophilic attack.[16]
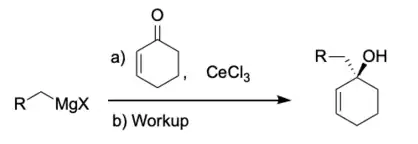
Organoceruim reagents are usually made by transmetalation from a Grignard reagent or organolithium reagent.[17]

Organocuprate Reagents (Gilman Reagents)
Organocuprates are a type of Grignard reagent that preferentially does 1,4 addition. Since conjugate additions are slower than 1,2 addition, Lewis Acids can be used to speed up a reaction.
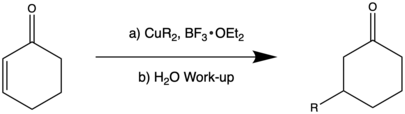
See also

References
- 1 2 Smith, Michael B.; March, Jerry (2007), Advanced Organic Chemistry: Reactions, Mechanisms, and Structure (6th ed.), New York: Wiley-Interscience, ISBN 978-0-471-72091-1
- ↑ Chapter 19: Carboxylic Acids. Organic Chemistry 4e Carey. mhhe.com
- 1 2 3 Ouellette, Robert J.; Rawn, J. David (January 1, 2014), Ouellette, Robert J.; Rawn, J. David (eds.), "15 - Alcohols: Reactions and Synthesis", Organic Chemistry, Boston: Elsevier, pp. 491–534, doi:10.1016/b978-0-12-800780-8.00015-2, ISBN 978-0-12-800780-8, retrieved November 6, 2023
- ↑ Shirley, D. A. (1954). "The Synthesis of Ketones from Acid Halides and Organometallic Compounds of Magnesium, Zinc, and Cadmium". Org. React. 8: 28–58.
- ↑ Huryn, D. M. (1991). "Carbanions of Alkali and Alkaline Earth Cations: (ii) Selectivity of Carbonyl Addition Reactions". In Trost, B. M.; Fleming, I. (eds.). Comprehensive Organic Synthesis, Volume 1: Additions to C—X π-Bonds, Part 1. Elsevier Science. pp. 49–75. doi:10.1016/B978-0-08-052349-1.00002-0. ISBN 978-0-08-052349-1.
- 1 2 texte, Académie des sciences (France) Auteur du (January 1, 1900). "Comptes rendus hebdomadaires des séances de l'Académie des sciences / publiés... par MM. les secrétaires perpétuels". Gallica. Retrieved April 23, 2023.
- ↑ Grignard, V. (1900). "Sur quelques nouvelles combinaisons organométaliques du magnésium et leur application à des synthèses d'alcools et d'hydrocabures". Compt. Rend. 130: 1322–25.
- 1 2 IUPAC. Compendium of Chemical Terminology, 2nd ed. (the "Gold Book"). Compiled by A. D. McNaught and A. Wilkinson. Blackwell Scientific Publications, Oxford (1997). ISBN 0-9678550-9-8. doi:10.1351/goldbook.
- ↑ Maruyama, K.; Katagiri, T. (1989). "Mechanism of the Grignard reaction". J. Phys. Org. Chem. 2 (3): 205–213. doi:10.1002/poc.610020303.
- ↑ Ashby, E. C.; Goel, A. B. (August 1981). "Direct evidence supporting a single electron transfer pathway in the reduction of ketones by primary, secondary, and tertiary Grignard reagents". Journal of the American Chemical Society. 103 (16): 4983–4985. doi:10.1021/ja00406a070. ISSN 0002-7863.
- ↑ Peltzer, Raphael Mathias; Gauss, Jürgen; Eisenstein, Odile; Cascella, Michele (February 12, 2020). "The Grignard Reaction – Unraveling a Chemical Puzzle". Journal of the American Chemical Society. 142 (6): 2984–2994. doi:10.1021/jacs.9b11829. hdl:10852/83918. ISSN 0002-7863. PMID 31951398. S2CID 210709021.
- ↑ Carey, Francis A. "Grignard reagent". Britannica.
- 1 2 "Grignard Reaction | The Merck Index Online". www.rsc.org. Retrieved April 23, 2023.
- 1 2 Ziegler, Dorothée S.; Wei, Baosheng; Knochel, Paul (February 21, 2019). "Improving the Halogen–Magnesium Exchange by using New Turbo‐Grignard Reagents". Chemistry – A European Journal. 25 (11): 2695–2703. doi:10.1002/chem.201803904. ISSN 0947-6539. PMID 30230067. S2CID 52295377.
- 1 2 Imamoto, Tsuneo; Sugiura, Yasushi (April 16, 1985). "Selective 1,2-addition of organocerium(III) reagents to α,β-unsaturated carbonyl compounds". Journal of Organometallic Chemistry. 285 (1): C21–C23. doi:10.1016/0022-328X(85)87395-2. ISSN 0022-328X.
- ↑ Berger, Tassilo; Lebon, Jakob; Maichle‐Mössmer, Cäcilia; Anwander, Reiner (July 5, 2021). "CeCl 3 / n ‐BuLi: Unraveling Imamoto's Organocerium Reagent". Angewandte Chemie International Edition. 60 (28): 15622–15631. doi:10.1002/anie.202103889. ISSN 1433-7851. PMC 8362106. PMID 33905590.
- ↑ Smith, Michael B. (January 1, 2017), Smith, Michael B. (ed.), "Chapter 11 - Carbon-Carbon Bond-Forming Reactions: Cyanide, Alkyne Anions, Grignard Reagents, and Organolithium Reagents", Organic Synthesis (Fourth Edition), Boston: Academic Press, pp. 547–603, doi:10.1016/b978-0-12-800720-4.00011-8, ISBN 978-0-12-800720-4, retrieved December 3, 2023